In July 2021, I wrote about my goal to reproduce the results of a paper using a high surface area carbon, loaded with elemental iodine, to create a practical Zinc-Iodine battery.
This was not successful because my material of choice – a GFE-1 cathode – did not absorb any iodine when placed in an iodine chamber at 55C for 10 minutes. This is because the cathode material is likely already passivated, as it has been in air for more than a year now, so activation would likely be necessary to get it to load with Iodine. Performing this process is outside my current technical possibilities (no ovens or vacuum chambers available).
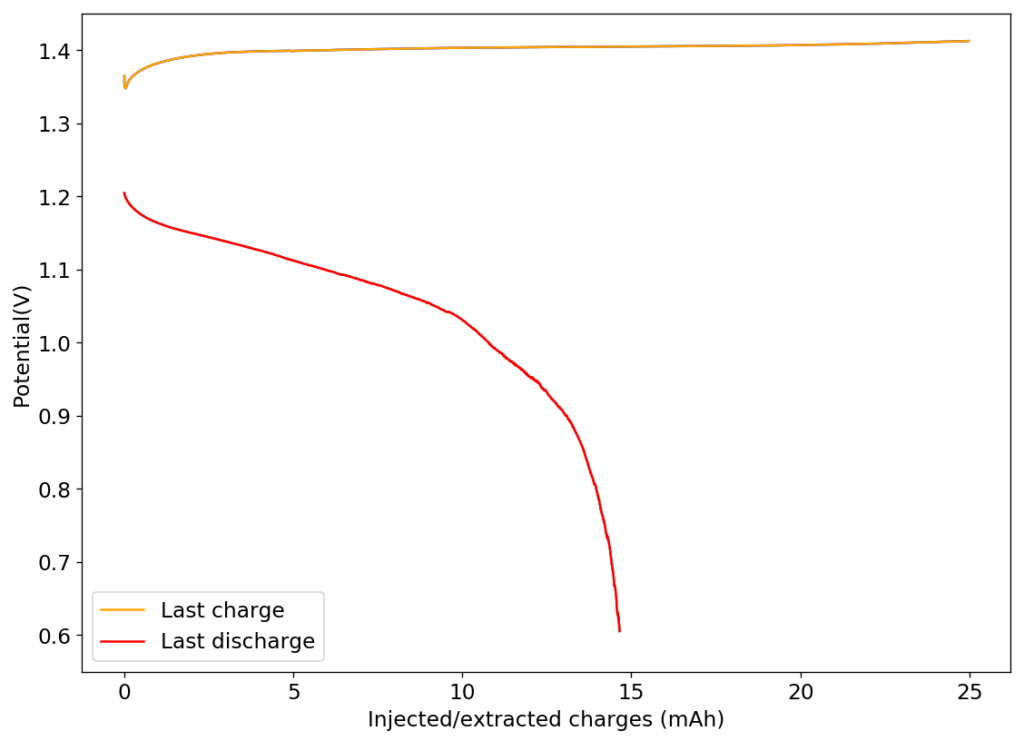
Instead of continuing down this path, I decided to create a battery using Zinc Iodide. This chemical is hard to get though, so I synthesized it from metallic Zinc and elemental Iodine. With the 2.5M solution ZnI2 I created – concentration determined by its density – I then proceeded to create batteries in my Swagelok cell configuration. The best result I got, where energy density was around 29 Wh/L, are shown above.
Despite the above, I was never able to achieve high CE or EE values, with the values for my batteries at >20Wh/L capacities being around 55% for CE and 35% for EE. Low coulombic efficiency happened because of diffusion of elemental iodine away from the cathode – through the formation and diffusion of I3– – as evidenced by the strong coloring of the fiber glass separators when taking the batteries apart. In a similar fashion to Zn-Br batteries, the elemental halogen diffuses and this inevitably lowers the CE, EE and increases the self-discharge of the battery. Even the high surface GFE-1 carbon felt, is just uncapable of holding to the halogen on its own.
Besides making most properties of the batteries worse, the diffusion eventually kills the battery, as so much reagent is lost per cycle that at some point the battery is simply unable to properly function, as any generated zinc is consumed by Iodine that reaches the anode.
I have tried several things so far to eliminate the problem. Thicker carbon material, more dilute ZnI2, TBABr as a complexing agent, none of these have worked, since I3– forms very efficiently under any excess of I– in the solution, which is inevitable as when the reaction starts there is ample iodide in solution. Adding TBAB does precipitate the TBAI3 salt, but this greatly increases the series resistance of the battery.
Is there anyway to stop the I2 from becoming I3– and migrating? Can we somehow plate elemental I2 at the cathode and avoid self-discharge and losses in CE and EE? Stay tuned for more research results.