For Zinc-Bromine batteries, energy density is a key characteristic since these batteries are bound to be used under circumstances where the specific energy (Wh/kg) is not as relevant as the amount of space taken by the batteries to store a given amount of energy (like utility level energy storage). Given this fact, I wanted to explore how hard I could push the capacity of a Zn-Br battery to try to maximize its energy density.
I built a cell with a GFE-1 cathode pretreated with a 50% TMPhABr solution, used 16 layers of fiberglass separator (total cell height 0.53cm, total area 1.29cm2, volume 0.68mL), used a 3M ZnBr2 + 20% PEG-200 solution to minimize dendrites as much as a I could. I then tried to charge the cell to 30mAh, see what sort of efficiencies I could get. I used a current of 5mA since the 20% PEG content and highly loaded GFE-1 cathode both substantially increased the internal resistance of the device.
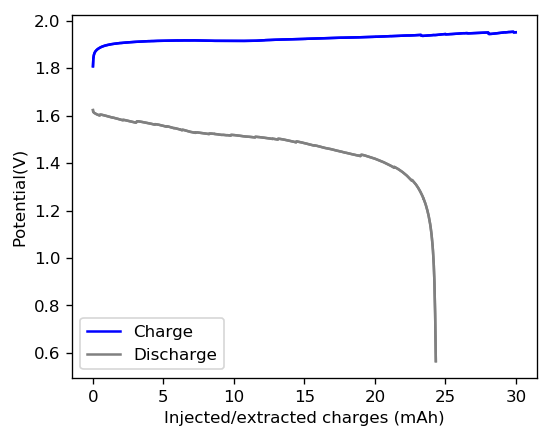
Given the results shown above, I was able to achieve an effective stored charge of 18.7mAh, which gives the cell an energy density of 43.76 Wh/L. This puts the battery above the values that are achieved for commercial Zinc-Bromine flow batteries (5.7–39 W·h/L). However not everything was as good as I thought, as the battery shorted during the second cycle due to the formation of Zinc dendrites. I was however very puzzled by the presence of Zinc dendrites at a 20% PEG-200 concentration, so I decided to open up the battery and peel the layers to see what was going on.
As you can see in the image below, zinc dendrites form predominantly across the first 3 layers of fiberglass separator, which means that the PEG-200 was indeed effective at preventing dendrite formation from advancing too much through the battery (without PEG-200 you would see a significant presence of dendrites all the way to the cathode). However there were some Zinc dendrites forming predominantly close to the edge of the battery and these progressed all the way to the cathode material, although it is very hard to see their presence without magnification within the last couple of layers.
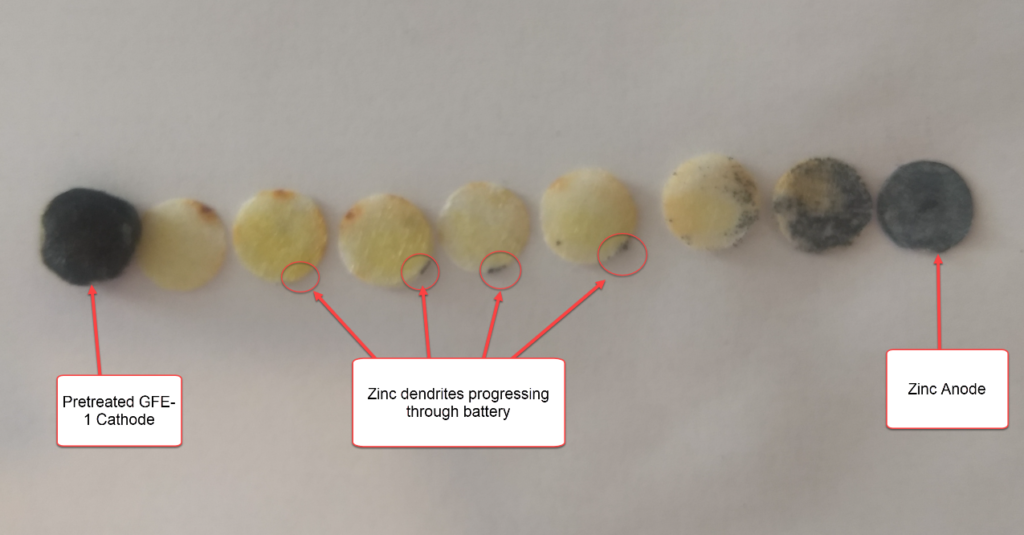
The fact that I am using a Zinc anode that is cut from a 0.2mm sheet with a perforator might have something to do with it, as the Zinc is bound to be extremely sharp at the edges – therefore high surface area – due to the cutting process. This is the perfect spot for the formation of dendrites and – due to the smaller amount of electrolyte at these points – could easily lead to the formation of dendrites moving through the battery, which is what we have observed. This also happened at a point where the Zn anode was particularly sharply cut, which further reinforces this hypothesis.
In order to see if the Zinc anode and the way it’s cut has a lot to do with this fact I have decided to repeat the above experiment using the graphite electrode as anode – without the presence of any Zinc anode – which should show if zinc dendrites are able to form all the way to the cathode in the presence of large concentrations of PEG-200. If Zinc dendrites do not form in this case, I will move to the use of graphite for the anode material from now on.