I have written several blog posts in the past about the potential use of propylene carbonate (PC) as a potential non-aqueous solvent in Zn-Br batteries. However, through my research I have now discovered that this solvent will not work in these devices as a cathode electrolyte, due to the way it interacts with the chemicals that are generated within the cell. In this post I will explain to you the experiments I did and why I reached this conclusion.
The idea with using PC was initially to completely replace the electrolyte within the cell. This was discarded right away due to ZnBr2 solubility issues and low conductivity issues of the constructed cells. The idea then evolved to using a tetrabutylammonium bromide (TBABr) saturated PC solution (PC-TBABr) as an organic layer in an inverted device, since this layer can rest on top of a Zn-Br solution and can even remain on top after mixing if the Zn-Br solution is concentrated enough (>4M).
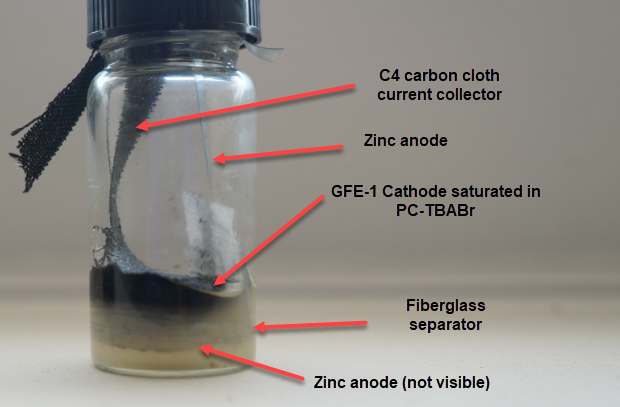
The organic layer is completely immiscible with the highly concentrated ZnBr2 layer and it was my hope that the TBABr3 produced in the cathode would be substantially more soluble in the PC-TBABr compared to the aqueous phase. Given that the PC phase is more than 50% TBABr, it seemed very likely that the produced perbromide would have a significantly higher affinity for the PC-TBABr.
To confirm whether this was happening, I constructed an inverted cell using a glass vial in order to be able to see what was going on (which you can see above). I placed a zinc anode at the bottom, used fiberglass as a separator and placed a GFE-1 cathode saturated with PC-TBABr on top using some C4 carbon cloth as a current collector. Before placing the GFE-1 cathode I filled the cell with a 4.2M ZnBr2 electrolyte, which makes the PC-TBABr remain less dense even after prolonged mixing. Since my objective was not to measure efficiencies with this device, but just to observe the chemical processes, I did not strive for a reproducible geometry.
After charging for a while with 3 charged AA batteries at more than 3V – just to make the process go fast – I noticed a lot of TBABr3 forming and precipitating within the cell. Sadly, the perbromide seems to form on the cathode and then immediately migrate out and into the aqueous phase. To my absolute surprise, the TBABr3 – which is more of a liquid rather than a solid – has higher affinity for the aqueous phase, although it doesn’t solubilize within it but rather forms a suspension with it.
I then proceeded to take the electrolyte out of the cell and perform an extraction using some additional PC-TBABr and surprisingly, all the perbromide stays in the aqueous phase after mixing and just refuses to get into the PC-TBABr. For this reason, PC is not going to work as a cathode electrolyte within the device, as the perbromide just exits it and never returns. This is probably why my devices trying to do this never seemed to work for too long.